Developing Adaptive Polymeric Materials through Stimulus-Responsive Chemistry
The Big Picture
In nature, biological materials display great adaptability to their environment. Cells produce, process and eliminate the materials required for an organism to live. They can also sense changes in their environment and adapt accordingly. They assemble into large and complex structures, such as organs and vascular networks, which allow for efficient mass and energy transport. These properties give biological materials great advantage in terms of efficiency and durability. In contrast, synthetic polymeric materials lack analogous adaptability. Significant effort has been devoted in designing novel chemical systems which impart synthetic polymers with self-reporting, self-healing and self-remodeling properties. Several reports have shown the use of heat, light, electricity, pH and pressure changes to mediate the identification of a failure in polymeric material, deposition of new material, or the formation of complex architectures and patterns. However, several challenges remain.
The Nitty Gritty
Morphogenesis for materials synthesis and design
Material microstructure is a key design element in advanced materials. Microstructure improves a material’s performance for compression, mass and heat transfer. Natural composites gain unmatched performance by continuously adapting their microstructure to their environment via reaction–diffusion (RD) sequences, a process termed morphogenesis. RD is the formation of spatial micropatterns by two reactive molecules diffusing at distinct, controlled rates. During lung development, for example, microstructures are morphed via RD. This action increases the efficiency of gas exchange by changing a micron-scale bud to a half-meter-long, hierarchical composite composed of thousands of identical micron-scale alveoli. Current RD-based synthesis of microstructures creates elegant patterns from microns to millimeters, but they are not a continuous process, are incapable of adaptation, and are limited to two dimensions. Still lacking in synthetic systems is the dynamic, continuous restructuring of 3D patterns that are the key principle that biological systems use to improve performance.
Our group is currently interested in conferring morphogenic properties to synthetic materials. In our previous work, we developed a basic system that allows the coupling of polymerization, depolymerization, and reaction diffusion to enable preliminary versions of synthetic morphogenesis. We have also developed artificial vascular networks that allow for efficient gas exchange. Currently, we are studying frontal polymerization as a means for coupling rapid and cost-effective materials fabrication with morphogenesis for polymer composite manufacturing.
1. Adaptive Materials Mediated by Mechanical Force
Biological materials such as bones, muscles and skin can adapt in response to mechanical stimuli by changing their composition and structure through a process called mechanotransduction. They efficiently convert mechanical stimuli into chemical signals that can drive reactions leading to structural changes, deposition and removal of material. Currently, synthetic polymeric materials mostly lack similar adaptability, resulting in mismatches in their physical properties and the need to replace them repeatedly. Recent years have seen a growing increase in reports of research groups using mechanically-responsive systems to generate novel polymerization methods. To date, most of the efforts have focused on improving chain growth mechanisms and either expanding the capabilities of current mechanophore reactions or finding new mediators of mechanically-driven radical generation. Far fewer experimental efforts have focused on expanding the chemical reactions possible with mechanically mediated polymerization or exploring the mechanism by which vibrations are converted to working electro-chemical reactivity.

2. Polymerization Strategies Mediated by Mechanical Force
Our group has pioneered the study of electrochemical reactions via the effect of piezoelectric oxide nanoparticles for conducting polymerization reactions under sonication conditions. So far, we have developed piezo-mediated copper ATRP, copper-catalyzed azide-alkyne cycloaddition, iron-mediated free radical polymerization and thiol-ene reactions. Our current goals include expanding the range of piezo-mediated chemistries, studying mechanistic aspects of the piezo-activation of chemical species and transitioning from solution-based polymerizations to gels and solids.


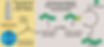